

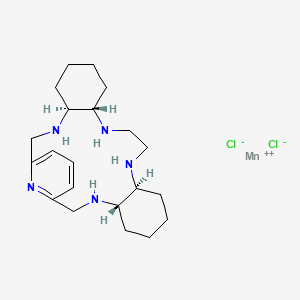
AVASOPASEM
Average: 518.83
Monoisotopic: 517.134397
Chemical FormulaC21H35Cl3MnN5
manganese(2+);(4S,9S,14S,19S)-3,10,13,20,26-pentazatetracyclo[20.3.1.04,9.014,19]hexacosa-1(26),22,24-triene;dichloride
-
Manganese, dichloro((4aS,13aS,17aS,21aS)-1,2,3,4,4a,5,6,12,13,13a,14,15,16,17,17a,18,19,20,21,21a-eicosahydro-7,11-nitrilo-7H-dibenzo(b,H)-5,13,18,21-tetraazacycloheptadecine-kappaN5,kappaN13,kappaN18,kappaN21,kappaN22)-, (pb-7-11-2344’3′)-
CAS 435327-40-5
- GC 4419
- M-40419
- SC-72325A
Avasopasem manganese, also known as GC4419, is a highly-selective small molecule mimetic of superoxide dismutase (SOD) being investigated for the reduction of radiation-induced severe oral mucositis.1,2 This drug has potential application for radiation-induced esophagitis and oral mucositis, in addition to being currently tested against COVID-19.
Avasopasem manganese is a superoxide dismutase mimetic that rapidly and selectively converts superoxide to hydrogen peroxide and oxygen in order to protect normal tissue from radiation therapy-induced damage.1 This drug is currently being investigated against oral mucositis, esophagitis, and COVID-19.
PATENT
https://patentscope.wipo.int/search/en/detail.jsf?docId=WO2018152353
Transition metal pentaaza 15-membered macrocyclic ring complexes having the macrocyclic ring system corresponding to Formula A have been shown to be effective in a number of animal and cell models of human disease, as well as in treatment of conditions afflicting human patients.

For example, in a rodent model of colitis, one such compound, GC4403, has been reported when administered by intraperitoneal (ip) injection to significantly reduce the injury to the colon of rats subjected to an experimental model of colitis (see Cuzzocrea et al., Europ. J. Pharmacol., 432, 79-89 (2001)).

GC4403 administered ip has also been reported to attenuate the radiation damage arising both in a clinically relevant hamster model of acute, radiation-induced oral mucositis (Murphy et al., Clin. Can. Res., 74(13), 4292 (2008)), and lethal total body irradiation of adult mice (Thompson et al., Free Radical Res., 44(5), 529-40 (2010)).
Similarly, another such compound, GC4419, administered ip has been shown to attenuate VEGFr inhibitor-induced pulmonary disease in a rat model (Tuder, et al., Am. J. Respir. Cell Mol. Biol., 29, 88-97 (2003)), and to increase the anti-tumor activity of anti-metabolite and anti-mitotic agents in mouse cancer models (see, e.g., WO2009/143454). In other studies, GC4419 and GC4403 have been shown to be similarly potent in various animal models of disease. Additionally, another such compound, GC4401, administered ip has been shown to provide protective effects in animal models of septic shock (S. Cuzzocrea, et. al., Crit. Care Med., 32(1 ), 157 (2004)) and pancreatitis (S. Cuzzocrea, et. al., Shock, 22(3), 254-61 (2004)).

[0003] Certain of these compounds have also been shown to possess potent anti-inflammatory activity and prevent oxidative damage in vivo. For example, GC4403 administered ip has been reported to inhibit inflammation in a rat model of inflammation (Salvemini, et.al., Science, 286, 304 (1999)), and prevent joint disease in a rat model of collagen-induced arthritis (Salvemini et al., Arthritis & Rheumatism, 44(12), 2009-2021 (2001)). In addition, these compounds have been reported to possess analgesic activity and to reduce inflammation and edema by systemic administration in the rat-paw carrageenan hyperalgesia model, see, e.g., U.S. Pat. No. 6,180,620.
[0004] Compounds of the class comprising GC4419 have also been shown to be safe and effective in the prevention and treatment of disease in human subjects. For example, GC4419 administered by intravenous (iV) infusion has been shown to reduce oral mucositis in head-and-neck cancer patients undergoing chemoradiation therapy (Anderson, C, Phase 1 Trial of Superoxide Dismutase (SOD) Mimetic GC4419 to Reduce Chemoradiotherapy (CRT)-lnduced Mucositis (OM) in Patients (pts) with Mouth or Oropharyngeal Carcinoma (OCC), Oral Mucositis Research Workshop,
MASCC/ISOO Annual Meeting on Supportive Care in Cancer, Copenhagen, Denmark (June 25, 2015)).
[0005] However, the administered dose when delivered systemically, for example by a parenteral route, can be limited in animal models and particularly in humans by systemic exposure and resulting toxicity that appears to be similar in nature among the pentaaza 15-membered macrocyclic ring dismutase mimetics of Formula A, particularly GC4403, GC4419, GC4401 and related compounds sharing the dicyclohexyl and pyridine motif in the macrocycle ring (e.g., compounds sharing the dicyclohexyl and pyridine motif generally include compounds according to Formula (I) below herein having W as an unsubstituted pyridine moiety, and wherein U and V are transcyclohexanyl fused rings) . For example, the maximum tolerated dose of GC4403 delivered as a 30-minute iv infusion in humans is 25 mg, or roughly 0.35 mg/kg in a 70-kg subject, and similar limitations exist for animal parenteral dosing. Thus, the efficacy of treatment of conditions such as local inflammatory disease or tissue damage of the alimentary canal may be limited when using systemic delivery of GC4403 and similar compounds.
[0006] In each of these compounds comprising the pentaaza 15-membered macrocyclic ring of Formula A, the five nitrogens contained in the macrocyclic ring each form a coordinate covalent bond with the manganese (or other transition metal coordinated by the macrocycle) at the center of the molecule. Additionally, manganese (or other appropriate transition metal coordinated with the macrocycle) forms coordinate covalent bonds with “axial ligands” in positions perpendicular to the roughly planar macrocycle. Such coordinate covalent bonds are characterized by an available “free” electron pair on a ligand forming a bond to a transition metal via donation and sharing of the electron pair thus forming a two-electron bond between the metal and the donor atom of the ligand (Cotton, F.A. & G. Wilkinson, Advanced Inorganic Chemistry, Chapter 5, “Coordination Compounds”, 2nd revised edn., Interscience Publishers, p.139 (1966); lUPAC Gold Book, online version http://goldbook.iupac.org/C01329.html). The coordinate covalent nature of the bonds between manganese (or other such appropriate transition metal) and the five macrocyclic ring nitrogens and between manganese (or other such transition metal) and each of the two chloro axial ligands is evidenced, for example, by the “single crystal” X-ray crystal structure of GC4403 (Fig. 11 ) and GC4419 (Fig. 12).
[0007] Coordination compounds contrast with ionic compounds, for example, salts, where in the solid state the forces between anions and cations are strictly coulombic electrostatic forces of attraction between ions of opposite charge. Thus, in salts, discrete cations and anions provide the force to maintain the solid state structure; e.g., such as the chloride ion and the sodium ion in a typical salt such as sodium chloride (Cotton, F.A. & G. Wilkinson, Advanced Inorganic Chemistry, Chapter 5, “The Nature of Ionic Substances”, 2nd revised edn., Interscience Publishers, pp. 35-36, 45-49 (1966).
[0008] Although pentaaza 15-membered macrocyclic ring complexes have been disclosed in the literature for a number of anti-inflammatory indications, the representative disclosures discussed above illustrate that such compounds are generally administered by intraperitoneal (ip) or intravenous (iv) injection to potentiate systemic bioavailability. Local (e.g. topical) administration has been reported as ineffective in animal models of inflammatory disease, particularly when measured against the efficacy of systemic administration methods (Murphy et al., Clin. Can. Res., 74(13), 4292 (2008); WO 2008/045559). One research group has reported inhibition of colonic tissue injury and neutrophil accumulation by intracolonic administration of a prototype pentaaza macrocycle superoxide dismutase mimetic (MnPAM) (having a different structure from GC4403), though that disclosure neither addresses systemic bioavailability of the compounds described therein, nor explore limitations resulting from systemic bioavailability impacting safety and/or efficacy of that specific compound (Weiss et al., J. Biol. Chem., 271(42): 26149-26156 (1996); Weiss, R. and Riley, D., Drugs Future, 21 (4): 383-389 (1996)).
[0009] Aspects of the present disclosure provide for formulations of pentaaza macrocyclic ring complexes of the class comprising GC4419, GC4403, and GC4401 that exhibit limited systemic bioavailability when administered orally (e.g. less than 20%, less than 15%, and even less than 10% bioavailability when dosed in appropriate oil-based formulations; see Table 1 and when combined with other formulations even less than 5%, and even less than 1%; see Example 28). In general, drug absorption from the gastrointestinal tract occurs via passive uptake so that absorption is favored when the drug is in a non-ionized (neutral) and lipophilic form. See, e.g., Goodman & Gilman’s: The Pharmacological Basis of Therapeutics, Ninth Edition, p. 5-9 (1996). Without wishing to be limited to any particular theory, this is also believed to be the case for this class of compounds, as exemplified by GC4403, where the axial ligands are both chloro moieties forming a coordinate covalent bond to the manganese and a neutral complex results:

The Mn(ll) pentaaza macrocyclic ring dichloro complexes, such as GC4419, GC4401, GC4444, and GC4403 (structures shown below) were synthesized using literature procedures. For GC4403 the chiral R,R-diaminocyclohexane is utilized as starting material,2 whereas for GC4419, the mirror-image enantiomer of GC4403, the chiral S,S-diaminocyclohexane is utilized instead.3,4 The remainder of the synthesis of GC4419 can be identical in all respects to the method published for GC4403.2 The synthesis of the GC4401 complex was reported previously in reference 5.

[00213] The synthesis of GC4444 which contains the additional 11-R-Methyl substituent generating a fifth chiral center on carbon (and is also derived from R,R-diaminocyclohexane) is made from the corresponding chiral tetraamine whose synthesis was published in reference 6 as Example 5C.
Syntheses of Axial Ligand Derivatives
[00214] The same Mn(II) pentaaza macrocyclic ring dichloro complexes (GC4419, GC4403, GC4444 and GC4401 ) were also used as the starting material precursors for the syntheses of other axial ligand bound derivatives using a generic synthesis scheme in which a large excess of a salt of an anion is used to displace the chloro ligand thereby generating the new compound.
EXAMPLE 2
[00215] Synthesis of Manganese(ll)bis-acetato[(4aS,13aS,17aS,21aS)-1,2,3,4,48,5,6,12,13,13a,14,15,16,17, 17a,18,19,20,21,21a- Eicosahydro-11,7-nitrilo-7H-dibenzo[b,h][1,4,7,10] tetraazacycloheptadecine-KN5, κΝ13, κΝ18, κΝ21, κΝ22]-, [bis-Acetato (GC4419)]. GC4701

[00216] Using a 500-mL Erlenmeyer, 100 mL of deionized (“DI”) water was added to 5.3 g of GC4419; the mixture was stirred vigorously for 15-20 min, then sonicated for 5 min. The resulting light brownish suspension was filtered through a 10-20 μ fritted funnel (ca. 0.3 g of solid material remained in the funnel). The resulting clear solution was added into a sodium acetate solution (ca. 429 mmol, 21 equiv in 100 mL DI water) as a stream in one portion. No solid separated and the yellowish solution was stirred for 5 additional min. The solution was transferred to a separatory funnel and extracted (3 X 50 mL) with dichloromethane. The organic layers were separated, combined, and transferred back into a separatory funnel. The dichloromethane solution was back-extracted (2 X 50 mL) with aqueous sodium acetate (32 g/100 mL). The dichloromethane layer was dried over MgSO4 (ca. 10 g) for 30 min (w/stirring), filtered using a 10-20 μ fritted funnel, and the solution taken to dryness using a rotavap. To the yellow oily solid resulting from taking the solution to dryness was added methanol (50 mL). This solution was then again taken to dryness on the rotovap to yield a light yellow foam/glass. This material was dried in vacuo at room temperature for two days.
[00217] The isolated yellowish brittle (4.11 g, 75% yield based on GC4419) was analyzed by HPLC and showed a purity of 99.7% and elemental analysis showed 0.98 wt. % residual chlorine. The elemental analysis is consistent with the expected bis-(acetato) structure C25H41MnN5O4●2H2O. Anal Cal’d: C, 53.00% ; H, 8.01 %; N, 12.36%, and Mn, 9.70%. Anal Found: C, 53.10% ; H, 8.34% ; Mn, 9.86%, N, 12.56%, and CI (as total halogen content), 0.98 wt. %.
Patent
WO 2002071054
https://patents.google.com/patent/WO2002071054A1/en
Superoxide dismutase (SOD) enzymes are enzymes that catalyze the dismutation of the free radical superoxide, the one-electron reduction product of molecular oxygen. The dismutation of the free radical superoxide involves the conversion of this one-electron reduction product of molecular oxygen to the nonradical molecular oxygen. Superoxide dismutase enzymes are a class of oxidoreductases which contain either Cu/Zn, Fe, or Mn at the active site. Superoxide dismutase (SOD) mimetic compounds are low molecular weight catalysts which mimic the natural enzyme function of the superoxide dismutase enzymes. Thus, superoxide dismutase mimetic compounds also catalyze the conversion of superoxide into oxygen and hydrogen peroxide, rapidly eliminating the harmful biologically generated superoxide species that are believed to contribute to tissue pathology in a number of diseases and disorders. These diseases and disorders include reperfusion diseases, such as those following myocardial infarct or stroke, inflammatory disorders such as arthritis, and neurological disorders such as Parkinson’s disease. Chem Reviews, 1999 vol 99, No. 9, 2573-2587.
Superoxide dismutase mimetic compounds possess several advantages over the superoxide dismutase enzymes themselves in that their chemical properties can be altered to enhance stability, activity and biodistribution while still possessing the ability to dismutase the harmful superoxide. Superoxide dismutase mimetic compounds have generated intense interest and have been the focus of considerable efforts to develop them as a therapeutic agent for the treatment of a wide range of diseases and disorders, including reperfusion injury, ischemic myocardium post-ischemic neuropathies, inflammation, organ transplantation and radiation induced injury. Most of the superoxide dismutase mimics currently being developed as therapeutic agents are synthetic low molecular weight manganese-based superoxide dismutase mimetic compounds. Chem Reviews, 2576. Superoxide dismutase mimetic compounds are metal complexes in which the metal can coordinate axial ligands. Examples of such metal complexes include, but are not limited to, complexes of the metals Mn and Fe. Many of the complexes of the metals Mn and Fe do not possess superoxide dismutase activity but possess properties that enable them to be put to other therapeutic and diagnostic uses. These therapeutic and diagnostic uses include MRI imaging enhancement agents, peroxynitrite decomposition catalysts, and catalase mimics. These metal complexes, however, share the structural similarity of possessing a metal that can coordinate exchangeable ligands. These metal complexes exist in water as a mixture of species in which various ligands are possible. An illustration of such a mixture is provided by M40403 , a Mn(π) complex of a nitrogen-containing fifteen membered macrocyclic ligand, shown in Scheme 1. One of the forms for this metal complex is the dichloro complex, which when dissolved in water another form is generated where one of the chloride anions immediately dissociates from the metal generating the [Mn(Cl)(aquo)]+ complex. The problem in aqueous solvent systems or any solvent which has a potential donor atom is that there are a variety of potential ligands available to coordinate axially to the Mn(π) ion of the complex, hi conducting an analysis of a sample containing a metal complex by high performance liquid chromatography (HPLC) the chromatogram tends to be very broad and unresolved due to the presence of the various species of complexes, as shown in Scheme 1. This phenomena makes the identification and quantification of metal complexes by standard HPLC techniques quite difficult. Therefore, in light of the developing roles of metal complexes as therapeutics in the treatment of various disorders and diagnostic agents, a substantial need exists for an effective and workable high performance liquid chromatography method for analyzing metal complexes.
Scheme 1
An additional complication which exists is the issue of the acid stability of the metal complex. As the pH decreases, the rate at which the complex becomes protonated and experiences instability increases. This presents particular problems for the use of HPLC as a method of detection and quantification of the metal complexes because the mobile phase used for reverse phase HPLC frequently contains mixtures of organic solvents and water in various combinations with trifluoroacetic acid. The trifluoroacetic acid is commonly present between about 0.1 to about 0.5% by weight. The presence of the trifluoroacetic acid causes the complex to dissociate. This dissociation destroys the potential of any such method to be used for release testing for purity. Furthermore, the trifluoroacetate anion causes the formation of some of the trifluoroacetato complex which could possess a different retention time from the chloro complexes thus, confusing the chromatography. Thus, the phenomenon of ligand exchange, coupled with the acid instability of the metal complexes, provides considerable challenges to the effort to detect and quantify metal complexes using HPLC. These challenges and needs have surprisingly been met by the invention described below.
Analytical HPLC is a powerful method to obtain information about a sample compound including information regarding identification, quantification and resolution of a compound. HPLC has been used particularly for the analysis of larger compounds and for the analysis of inorganic ions for which liquid chromatography is unsuitable. Skoog, D.A., West, M.A., Analytical Chemistry, 1986, p. 520. As an analytical tool HPLC takes advantage of the differences in affinity that a particular compound of interest has for the stationary phase and the mobile phase (the solvent being continuously applied to the column). Those compounds having stronger interactions with the mobile phase than with the stationary phase will elute from the column faster and thus have a shorter retention time. The mobile phase can be altered in order to manipulate the interactions of the target compound and the stationary phase. In normal-phase HPLC the stationary phase is polar, such as silica, and the mobile phase is a nonpolar solvent such as hexane or isopropyl ether. In reversed- phase HPLC the stationary phase is non-polar, often a hydrocarbon, and the mobile phase is a relatively polar solvent. Since 1974 when reversed-phase packing materials became commercially available, the number of applications for reversed- phase HPLC has grown, and reversed- phase HPLC is now the most widely used type of HPLC. Reversed-phase HPLC’s popularity can be attributed to its ability to separate a wide variety of organic compounds. Reversed-phase chromatography is especially useful in separating the related components of reaction mixtures, and therefore is a useful analytical tool for determining the various compounds produced by reactions. To create a non-polar stationary phase silica or synthetic polymer based adsorbents are modified with hydrocarbons. The most popular bonded phases are Cl, C4, C8 and C18. Silica based adsorbents modified with trimethylchlorosilane (Cl) and butyldimethylchlorosilane (C4) have a few applications in HPLC, mainly for protein separation or purification. These adsorbents show significant polar interactions. Octyl (C8) and octadecyl (C18) modified adsorbents are the most widely used silica based adsorbents, with almost 80% of all HPLC separations being developed with these adsorbents.
The most important parameter in reversed-phase HPLC is the mobile phase. The type of mobile phase employed in the HPLC will have a significant effect on the retention of the analytes in the sample, and varying the composition of the mobile phase allows the chromatographer to adjust the retention times of target components in the mixture to desired values. This ability provides the HPLC method with flexibility. The mobile phase in reversed-phase chromatography has to be polar and it also has to provide reasonable competition for the adsorption sites for the analyte molecules. Solvents that are commonly employed as eluent components in reversed-phase HPLC are acetonitrile, dioxane, ethanol, methanol, isopropanol, tetrahydrofuran, and water. In reversed phase HPLC of high molecular weight biological compounds, the solvents acetonitrile, isopropanol or propanol are most frequently used. Popular additives to the mobile phase for the improvement of resolution include mixtures of phosphoric acid and amines and periϊuorinated carboxylic acids, especially trifluoroacetic acid (TFA). HPLC exploits the differences in affinity that a particular compound of interest has for the stationary phase and the mobile phase. This phenomenon can be utilized to separate compounds based on the differences in their physical properties. Thus, HPLC can be used to separate stereoisomers, diastereomers, enantiomers, mirror image stereoisomers, and impurities. Stereoisomers are those molecules which differ from each other only in the way their atoms are oriented in space. The particular arrangement of atoms that characterize a particular stereoisomer is known as its optical configuration, specified by known sequencing rules as, for example, either + or – (also D or L) and/or R or S. Stereoisomers are generally classified as two types, enantiomers or diastereomers. Enantiomers are stereoisomers which are mirror-images of each other. Enantiomers can be further classified as mirror-image stereoisomers that cannot be superimposed on each other and mirror-image stereoisomers that can be superimposed on each other. Mirror- image stereoisomers that can be superimposed on each other are known as meso compounds. Diastereomers are stereoisomers that are not mirror images of each other. Diastereomers have different physical properties such as melting points, boiling points, solubilities in a given solvent, densities, refractive indices, etc. Diastereomers can usually be readily separated from each other by conventional methods, such as fractional distillation, fractional crystallization, or chromatography, including HPLC.
Enantiomers, however, present special challenges because their physical properties are identical. They generally cannot be separated by conventional methods, especially if they are in the form of a racemic mixture. Thus, they cannot be separated by fractional distillation because their boiling points are identical and they cannot be separated by fractional crystallization because their solubilites are identical (unless the solvent is optically active). They also cannot be separated by conventional chromatography such as HPLC because (unless the adsorbent is optically active) they are held equally onto the adsorbent. HPLC methods employing chiral stationary phases are a very common approach to the separation of enantiomers. To be able to separate racemic mixtures of stereoisomers, the chiral phase has to form a diastereomeric complex with one of the isomers, or has to have some other type of stereospecific interaction. The exact mechanism of chiral recognition is not yet completely understood. In reversed-phase
HPLC a common type of chiral bonded phase is chiral cavity phases.
The ability to be able to separate diastereomers and enantiomers by HPLC is a useful ability in evaluating the success of synthetic schemes. It is often desirable to separate stereoisomers as a means of evaluating the enantiomeric purity of production samples. All references listed herein are hereby incorporated by reference in their entirety
Examples 1 (traditional mobile phase) and 2 (mobile phase containing excess of salt of a coordinating anion).
+X“
Scheme 2 Any metal complex possessing a metal that is capable of coordinating a monodentate ligand can be used in the present invention. Examples of such metal complexes include, but are not limited to, complexes of the metals Mn and Fe. The metal complexes of the invention preferably have therapeutic and diagnostic utilities. These therapeutic and diagnostic utilities include, but are not limited to, use as superoxide dismutase mimetic compounds, MRI imaging enhancement agents, peroxynitrite decomposition catalysts, and catalase mimics. The preferred metal complexes for use in the invention are superoxide dismutase mimetic compounds. Examples of such superoxide dismutase mimetic compounds include, but are not limited to, the following complexes of the metals Mn and Fe. Iron based superoxide dismutase mimetics include, but are not limited to, Fera(salen) complexes, Fera(l,4,7,10,13-pentaazacyclopentadecane) derivatives and Feffl(porphyrinato) complexes. Manganese based superoxide dismutase mimetic compounds include, but are not limited to, metal complexes containing manganese(π) or manganese(m). Examples of manganese based superoxide dismutase mimetic compounds include Mnm(porphyrinato) complexes, Mnffl(salen) complexes, and Mnπ(l ,4,7, 10, 13-pentaazacyclopentadecane) derivatives. Mnπ(l ,4,7, 10,13- pentaazacyclopentadecane) derivatives are more preferred for use in the invention. Examples of Mnπ(l,4,7,10,13-pentaazacyclopentadecane) derivatives preferred for use in the invention include, but are not limited to, M40403 and M40401, as shown in Scheme 3 below.
Furthermore, stereoisomers of all of the above metal complexes can be used in the process of the present invention. Diastereomers of the same metal complexes can also be detected and separated by the method of the present invention. As it is often desirable to separate stereoisomers as a means of evaluating the chemical and optical purity of production samples, the metal complexes can also comprise products of a reaction stream. Enantiomers of any of the metal complexes referenced above can be used in the chiral HPLC method of the invention for the separation of enantiomers of a metal complex.
M40484
Scheme 3
The ligand is a coordinating anion that binds to the metal cation of the metal complex. The coordinating anion can serve as an axial ligand for a superoxide dismutase mimetic compound. Examples of such anions include, but are not limited to, chloride anions, thiocyanate anions, stearate anions, acetate anions, trifluoroacetate anions, carboxylate anions, formate anions, or azide anions. Preferred anions include chloride anions, thiocyanate anions, and formate anions. More preferred anions are chloride anions. The more preferred anions in the chiral HPLC embodiment of the invention are thiocyanate anions. When present in an excess, the thiocyanate anions bind to the coordinating metal of the complexes preferentially to the chloride anions. An excess of thiocyanate anions will produce the bis(thiocyanato) complexes of M40403 and M40419 as shown in Scheme 4.
M40419 M40419-(SCN)2
Scheme 4
An example of the use of the acetate anion as the coordinating anion with M40403 is shown in Scheme 5 below. Scheme 6 illustrates the use of the formate anion as the coordinating anion with M40403.
M40403 M40403-(OAc)2
Scheme 5
M40403 M40403-(Formate)2
Scheme 6
The coordinating anion is supplied by a salt of the coordinating anion. Salts of the chloride anion include, but are not limited to, sodium chloride, lithium chloride, potassium chloride, ammonium chloride, or tetraalkylammonium chloride. Preferred salts of the chloride anion include sodium chloride, lithium chloride and tetrabutylammonium chloride. Salts of the thiocyanate anion include, but are not limited to, sodium thiocyanate, potassium thiocyanate, ammonium thiocyanate, or lithium thiocyanate. Preferred salts of the thiocyanate anion include sodium thiocyanate and potassium thiocyanate. Salts of the acetate anion include, but are not limited to, potassium acetate, sodium acetate, ammonium acetate, ammonium trifluoroacetate and lithium acetate. Preferred salts of the acetate anion include ammonium acetate. Salts of the formate anion include, but are not limited to, potassium formate, sodium formate, ammonium formate and lithium formate. Preferred salts of the formate anion include ammonium formate. Salts of the cyanate anion include but are not limited to, sodium cyanate, potassium cyanate, or ammonium cyanate. Salts of the carboxylate anion include, but are not limited to, potassium carboxylate, ammonium carboxylate and sodium carboxylate. Salts of the stearate anion include, but are not limited to, lithium stearate and sodium stearate. Salts of the azide anion include, but are not limited to, sodium azide, potassium azide, and lithium azide. The salt added to the mobile phase can also be a mixture of any of these salts. Examples include a mixture of tetrabutylammonium chloride and lithium chloride.
EXAMPLES
Experimental For Examples 1-8 Chemicals, Solvents and Materials
All solvents used in the study were HPLC grade or equivalent. All chemicals were ACS reagent grade or equivalent.
HPLC System and Data Analysis
The HPLC chromatography was performed using a Gilson system (Model 306 pump, Model 155 UN-V detector, Model 215 liquid handler, Unipoint Software,
Win98), a Narian system (Model 310 pump, Model 340 UN-N detector, Model 410 autosampler Star Workstation, Win98) or SSI system (Acuflow Series IN pump, Acutect 500 UV-N detector, Alcott Model 718 autosampler, HP Model 3395 integrator).
Example 1
HPLC Analysis of M40403 using Method 1
M40403 Method 1: Analytical Column: Waters YMC ODS-AQ S5 120A (4.6 x 50 mm); System A: 0.1% trifluoroacetic acid in H2O; System B: 0.08% trifluoroacetic acid in acetonitrile; Gradient: 10-50% system B over 10 min; Flow rate: 3ml/min; Detector wavelength: 265. Injected 20 μl of stock solution of M40403 prepared by dissolving 1 mg in 1 ml of water and diluting with 1 ml of system A. The HPLC chromatogram of M40403 using method 1 is shown in Figure 1. Example 2 HPLC Analysis of M40403 using Method 2
Method 2: Analytical Column: Waters YMC 9DS-AQ S5 12θΛ (4.6 x 50 MM); System A: 0.5 N aqueous NaCl; System B: 1 :4 water/CH3CN; Gradient: 10-50% system B over 9 min; Flow rate: 3mL/min; Detector wavelength: 265 nm. Injected 20 μl of stock solution of M40403 prepared by dissolving 1 mg in 1 ml of system A. The HPLC chromatogram of M40403 using method 2 is shown in Figure 2.
Example 3 HPLC Analysis of M40403 using Method 3
Method 3: Analytical Column: Waters Symmetry Shield RP18, 5 μm, 250 x 4.6 mm;
Mobile Phase: Acetonitrile: 0.125 M Tetrabutylammonium Chloride in water (pH 6.5), 5%: 95% H20(v/v); Flow rate: 1 mL/min; Detection wavelength: 265nm. Injected 20 μl of stock solution of M40403 prepared by dissolving 1 mg in 1 ml of mobile phase. The HPLC chromatogram of M40403 using method 3 is shown in Figure 3.
The HPLC chromatogram of M40403 and related compounds using method 3 is shown in Figure 3a. Method 3 allows a separation of M40402 (bisimine of M40403), M40414 (monoimine of M40403) and M40475 (free ligand of M40403) (see chromatogram in Figure 3a).
Example 4
HPLC Analysis of M40403 using Method 4
Method 4: Analytical Column: Waters Symmetry Shield RP18, 5 μm, 250 x 4.6 mm; Mobile Phase: Acetonitrile: 0.125 M Tetrabutylammonium Chloride and 0.5 M LiCl in water (pH 6.5), 5%: 95% H20 (v/v); Flow rate: lmL/min; Detection wavelength: 265 nm. Injected 20 μl of stock solution of M40403 prepared by dissolving 1 mg in 1 ml of system A. The HPLC chromatogram of M40403 using method 4 is shown in Figure 4.
The HPLC chromatogram of M40403 and related compounds using method 4 is shown in Figure 4a. Method 4 allows a separation of M40402 (bisimine of M40403), M40414 (monoimine of M40403) and M40475 (free ligand of M40403) and all diastereomers of M40403 (see chromatogram in Figure 4a).
Example 5 HPLC Analysis of M40401 using Method 1
M40401 Method 1: Analytical Column: Waters YMC ODS-AQ S5 120A (4.6 x 50 mm); System A: 0.1 % trifluoroacetic acid in H2O; System B: 0.08% trifluoroacetic acid in acetonitrile; Gradient: 10-50% system B over 10 min; Flow rate: 3ml/min; Detector wavelength: 265. Injected 20 μl of stock solution of M40401 prepared by dissolving 1 mg in 1 ml of water and diluting with 1 ml of system A. The HPLC chromatogram of M40401 using method 1 is shown in Figure 5.
Example 6 HPLC with various NaCl concentrations:
An HPLC was taken of M40401 with various concentrations of NaCl.
Analytical Column: Waters YMC 9DS-AQ S5 120 A (4.6 x 50 mm);
System A: (A) H2O (no NaCl) ; (B) 0.01 M NaCl in water; (C) 0.5 M NaCl in water;
System B: acetonitrile; Gradient: 0-100% system B over 10 min; Flow: 3 ml/min;
Detector wavelength: 265 nm. Injected 20 μl of stock solution of M40401 prepared by dissolving 1 mg in 1 ml of system A. The HPLC chromatogram of M40401 using various NaCl concentrations is shown in Figure 6. Example 7 HPLC Analysis of M40401 using Method 2
Method 2: Analytical Column: Waters YMC ODS-AQ S5 12θΛ (4.6 x 50 MM); System A: 0.5 N aqueous NaCl; System B: 1 :4 water/CH3CN; Gradient 1 : 10-50% system B over 9 min; Flow rate: 3 mL/min; Detector wavelength: 265 nm. Injected 20 μl of stock solution of M40403 prepared by dissolving 1 mg in 1 ml of system A.
The HPLC chromatogram of M40401 using method 2 is shown in Figure 7. Method 2 allows a separation of M40472 (bisimine of M40401), M40473 (monoimine of M40401), free ligand of M40403 and two isomers of M40401 (M40406, M40404).
Example 8
HPLC Analysis of M40401 using Method 3
Method 3: Analytical Column: Waters Symmetry Shield RP18, 5 m, 250 4.6 mm; Mobile Phase: Acetonitrile: 0.125 M Tetrabutylammom‘um Chloride in H20 (pH 6.5), 5: 95%) H20 (v/v); Flow rate: lmL/min; Detection wavelength: 265 nm. The HPLC chromatogram of M40401 using method 3 is shown in Figure 8.
Method 3 allows a separation of M40472 (bisimine of M40401), M40473 (monoimine of M40401), free ligand of M40403 and two isomers of M40401 (M40406, M40404).
Example 9 HPLC Analysis of M40401 using Method 4
Method 4: Analytical Column: Waters Symmetry Shield RP18, 5 μm, 250 x 4.6 mm;
Mobile Phase: Acetonitrile: 0.125 M Tetrabutylammonium Chloride and 0.5 M LiCl in water (pH 6.5), 5: 95%> H2O (v/v); Flow rate: 1 mL/min; Detection wavelength: 265 nm; Injected 20 μl of stock solution of M40401 prepared by dissolving 1 mg in 1 ml of a mobile phase. The HPLC chromatogram of M40401 using method 4 is shown in Figure 9.
The HPLC chromatogram of M40401 and related compounds using method 4 is shown in Figure 9a. Method 4 allows a separation of M40472 (bisimine of M40401), M40473 (monoimine of M40401), free ligand of M40403 and two isomers of M40401 (M40406, M40404). Example 10
HPLC of M40403-(HCOO“)2 Using Formate Anion
An HPLC of M40403 employing the formate anion was taken. Analytical Column: Waters YMC 9DS-AQ S5 120 A (4.6 x 50 mm); System A: 0.025 M ammonium formate in water; System B: 1 : 4 = 0.125 M ammonium formate in water/ acetonitrile; Gradient: 0-100% system B over 10 min; Flow: 3 ml/min;
Detector wavelength: 265 nm. Injected 20 μl of stock solution of M40403-(Formate)2 prepared by dissolving 1 mg in 1 ml of system A. The HPLC chromatogram of M40403-(HCOO“)2 is shown in Figure 10.
Example 11 HPLC of M40403-(OAc)2 Using Acetate Anion
An HPLC of M40403 employing the acetate anion was taken.
Analytical Column: Waters YMC 9DS-AQ S5 120 A (4.6 x 50 mm); System A: 0.025 M ammonium acetate in water; System B: 1: 4 = 0.125 M ammonium acetate in water/ acetonitrile; Gradient: 0-100% system B over 10 min; Flow: 3 ml/min;
Detector wavelength: 265 nm. Injected 20 μl of stock solution of M40403-(OAc)2 prepared by dissolving 1 mg in 1 ml of system A. The HPLC chromatogram of M40403 -(OAc)2 is shown in Figure 11.
Example 12
An HPLC method to separate the diastereomers of superoxide dismutase mimetic compound M40403. Four stereoisomer mixtures were prepared (Part A) as shown in Schemes 5-9 and then separated (Part B) via reversed-phase high performance liquid chromatography. Part A: Synthesis of Stereoisomers Of M40403
M40403 is synthesized from its single-isomer, tetra-amine precursor M40400 in the reaction shown in Scheme 7.
M40400 M40402
M40403
Scheme 7
The various stereoisomers of M40403 are synthesized from the various isomers of 1,2-diaminocyclohexane which provides the chiral carbon centers in M40403. The 1,2-diaminocyclohexane isomers used to prepare the R,R+R,S) M40403 stereoisomer mixture of Set 1 are shown in Scheme 6. Similarly, the 1,2-diaminocyclohexane isomers used to prepare the (R,R+S,S) M40403 stereoisomer mixture of Set 2 are shown in Scheme 7. The 1,2-diaminocyclohexane isomers used to prepare the (R,S+R,S) M40403 stereoisomer mixture of Set 3 are shown in Scheme 8. The 1,2- diaminocyclohexane isomers used to prepare the (S,S+R,S) M40403 stereoisomer mixture of Set 4 are shown in Scheme 9. As shown in Schemes 6-9 the M40403 diastereomers are prepared by template cyclization, followed by reduction with sodium borohydride.
Scheme 8
Scheme 9
Scheme 10
Scheme 11
Table 1
Part B: Separation of Stereoisomer Mixtures
Chemicals, Materials, and Methods
Tetrabutylammonium chloride hydrate (98%, 34,585-7) was purchased from Aldrich Chemical Company. Sodium chloride (99.6%, S-9888) was purchased from Sigma Chemical Company. All other solvents (HPLC-grade unless otherwise indicated) and reagents were purchased from Fisher Scientific and were of the finest grade available. The SymmetryShield® RP18 column (4.6 mm x 250 mm, 5 μm particle size) and its corresponding guard column were purchased from Waters Corporation. Reversed-Phase HPLC Experiments
Preparation of Standard Solutions
HPLC Mobile phased was an aqueous solution consisting of 0.125 M tetrabutylammonium chloride (TBAC) and 0.5 M LiCl, prepared by adding tetrabutylammonium chloride hydrate (36.99 g) and solid LiCl (21.2 g) to a 1 L volumetric flask, diluting to volume with Millipore water, and inverting the flask several times to obtain a homogeneous solution. The resulting solution was filtered through a 0.45 μm nylon filter prior to use. Mobile phase B was HPLC-grade acetonitrile. Samples of each diastereoisomer set for HPLC-UN analysis were prepared at concentrations of ~ 3.0 mg/mL in a 50:50 mixture of 0.5 M LiCl in MeOH:
PATENT
WO/2021/163397
SOLID STATE FORMS OF AVASOPASEM MANGANESE AND PROCESS FOR PREPARATION THEREOF
Avasopasem manganese (GC4419), has the following chemical structure:

[0003] Avasopasem manganese is a highly selective small molecule superoxide dismutase (SOD) mimetic which is being developed for the reduction of radiation-induced severe oral mucositis (SOM). The compound is described in U.S. Patent No. 8,263,568.
[0004] Polymorphism, the occurrence of different crystalline forms, is a property of some molecules and molecular complexes. A single molecule may give rise to a variety of polymorphs having distinct crystal structures and physical properties like melting point, thermal behaviors (e.g., measured by thermogravimetric analysis (“TGA”), or differential scanning calorimetry (“DSC”)), X-ray diffraction (XRD) pattern, infrared absorption fingerprint, and solid state (13C) NMR spectrum. One or more of these techniques may be used to distinguish different polymorphic forms of a compound.
[0005] Different salts and solid state forms (including solvated forms) of an active pharmaceutical ingredient may possess different properties. Such variations in the properties of different salts and solid state forms and solvates may provide a basis for improving formulation, for example, by facilitating better processing or handling characteristics, changing the
dissolution profile in a favorable direction, or improving stability (polymorph as well as chemical stability) and shelf-life. These variations in the properties of different salts and solid state forms may also offer improvements to the final dosage form, for instance, if they serve to improve bioavailability. Different salts and solid state forms and solvates of an active pharmaceutical ingredient may also give rise to a variety of polymorphs or crystalline forms, which may in turn provide additional opportunities to assess variations in the properties and characteristics of a solid active pharmaceutical ingredient.
[0006] Discovering new solid state forms and solvates of a pharmaceutical product may yield materials having desirable processing properties, such as ease of handling, ease of processing, storage stability, and ease of purification or as desirable intermediate crystal forms that facilitate conversion to other polymorphic forms. New solid state forms of a pharmaceutically useful compound can also provide an opportunity to improve the performance characteristics of a pharmaceutical product. It enlarges the repertoire of materials that a formulation scientist has available for formulation optimization, for example by providing a product with different properties, including a different crystal habit, higher crystallinity, or polymorphic stability, which may offer better processing or handling characteristics, improved dissolution profile, or improved shelf-life (chemical/physical stability). For at least these reasons, there is a need for additional solid state forms (including solvated forms) of Avasopasem manganese.
EXAMPLES
Preparation of starting materials
[00119] Avasopasem manganese can be prepared according to methods known from the literature, for example U.S. Patent No. 8,263,568. Alternatively, Avasopasem manganese can be prepared by the template method reported for the enantiomeric analogue GC4403, which has the formula:

GC4403 is disclosed in International Appl. No. WO 98/58636 (as compound SC-72325) and Riley, D.P, and Schall, O.F., Advances in Inorganic Chemistry (2007), 59, 233-263. Thus, GC4403 can be synthesized via the template route described in the literature using the chiral R,R-l,2-diamminocyclohexane [Salvemini, D., et ah, Science (1999), 286, 304-6 , and Aston, K, et al., Inorg. Chem. (2001), 40(8), 1779-89] Avasopasem manganese (GC4419) can be prepared by the same method except that the chiral R,R-l,2-diamminocyclohexane is replaced with S,S-1 ,2-diamminocyclohexane.
Example 1: Preparation of Avasopasem manganese Form AMI
[00120] Avasopasem manganese (0.1 grams) was dissolved in dichloromethane (0.5 ml) at 25-30°C in a test tube. The solution was filtered through 0.45 micron filter and the clear solution was subjected to slow solvent evaporation at 25°C by covering the tube with paraffin film with a pin hole. After, 2 days, the obtained solid was analyzed by XRD- Form AMI; as shown in Figure 1
- GlobeNewswire: Galera Therapeutics Announces Avasopasem Manganese Improved Markers of Chronic Kidney Disease in Patients Receiving Cisplatin [Link]
- Galera Therapeutics: AVASOPASEM (GC4419) [Link]
///////////AVASOPASEM, Avasopasem manganese, GC-4419, GC4419, GC 4419, M 40419, M40419; M-40419, SC 72325A, SC-72325A, SC72325A,
[Cl-].[Cl-].[Mn++].C1CC[C@@H]2NCC3=CC=CC(CN[C@H]4CCCC[C@@H]4NCCN[C@H]2C1)=N3